Aluminum oxide (Al2O3) has a high melting point because its atoms are strongly glued together with both ionic and covalent bonds. These bonds make it hard for the atoms to break apart, so you need a lot of heat to make it melt.
Further, that’s why it melts at very high temperatures, around 2,000 to 2,200 degrees Celsius (3,632 to 3,992 degrees Fahrenheit). This makes it great for uses where it has to withstand high heat, like in making things that can handle very hot conditions.
Here, you will find out more knowledge about it.
Chemical Structure of Aluminum Oxide
Aluminum Cations (Al3+):
- Aluminum (Al) is a metal that loses three electrons to form a cation (Al3+).
- Each aluminum ion has a 3+ charge due to the loss of three electrons.
- These cations are relatively small and positively charged.
Oxide Anions (O2-):
- Oxygen (O) is a non-metal that gains two electrons to form an anion (O2-).
- Each oxide ion has a 2- charge due to the gain of two electrons.
- Oxide ions are relatively large and negatively charged.
Ionic Bonds:
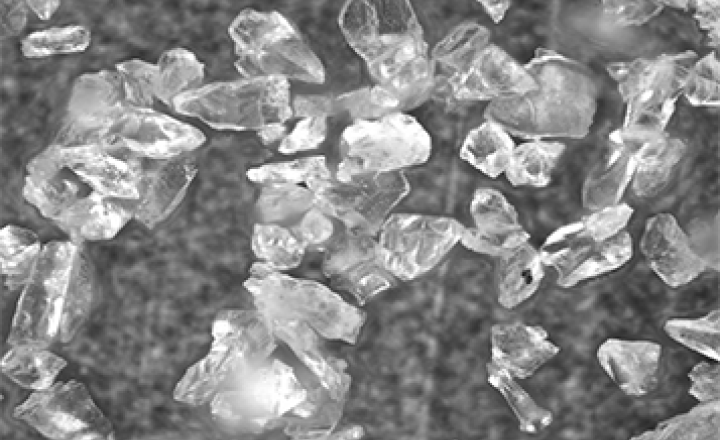
- It is held together by strong electrostatic forces of attraction between the positively charged aluminum cations and the negatively charged oxide anions.
- The ionic bonds are formed due to the transfer of electrons from aluminum to oxygen, resulting in the formation of Al3+ and O2- ions.
Crystal Lattice Structure:
- The aluminum cations (Al3+) and oxide anions (O2-) are arranged in a three-dimensional crystal lattice structure.
- This is repeated throughout the entire solid, creating a highly organized and stable arrangement.
Stoichiometry:
- It reflects the stoichiometry of the compound. It indicates that two aluminum cations combine with three oxide anions in a 2:3 ratio.
This ionic structure and strong electrostatic attraction between the oppositely charged ions are responsible for the high melting point and other unique properties of aluminum oxide. The strong ionic bonds make it difficult to break the crystal lattice, requiring a significant amount of energy to melt the substance or convert it into a liquid or molten state. Also, known for its hardness, thermal stability, and electrical insulating properties, which make it valuable in various industrial and scientific applications.
Strong Electrostatic Forces
Nature of Electric Charge:
Electric charge is a fundamental property of matter, and there are two types: positive and negative. Like charges (positive-positive or negative-negative) repel each other, while opposite charges (positive-negative) attract each other.
Coulomb’s Law:
Coulomb’s law is the fundamental equation that describes the electrostatic force between two charged particles. It is expressed as F = k * (q1 * q2) / r^2, where:
- F is the electrostatic force.
- k is the electrostatic constant.
- q1 and q2 are the magnitudes of the charges.
- r is the distance between the charges.
Ionic Compounds:
In ionic compounds such as salts, strong electrostatic forces are responsible for holding the positively charged ions (cations) and negatively charged ions (anions) together. The attraction between cations and anions creates the ionic bonds that give these compounds their characteristic properties, like high melting and boiling points.
Covalent Compounds:
Here electrons are shared between atoms. While covalent bonds also involve electrostatic forces, they are generally weaker than the ionic bonds found in ionic compounds. The strength of covalent bonds depends on the electronegativity of the atoms involved.
Van der Waals Forces:
including London dispersion forces, dipole-dipole interactions, and hydrogen bonding, are also types of electrostatic forces. They are responsible for intermolecular attractions between molecules and play a significant role in the physical properties of substances.
Applications:
Strong electrostatic forces are fundamental in various chemical reactions, molecular interactions, and the behavior of materials. They influence the stability of chemical compounds, the structure of molecules, the behavior of ions in electrolyte solutions, and the functioning of devices such as capacitors and transistors in electronics.
Distance and Charge:
The strength of electrostatic forces is inversely proportional to the square of the distance between the charged particles. That is, as the distance increases, the force decreases. Additionally, the magnitude of the charge directly affects the strength of the force, with larger charges leading to stronger electrostatic interactions.
Coordination Number and Ionic Radius
Coordination Number:
- Coordination number (CN) refers to the number of ions or atoms that immediately surround a central ion in a crystal lattice. In the context of ionic compounds, the central ion is typically an ion of one element (e.g., cation), and the surrounding ions are usually of the opposite charge (e.g., anions).
- For example, in sodium chloride (NaCl), which consists of sodium (Na+) cations and chloride (Cl-) anions, each sodium ion is surrounded by six chloride ions, and each chloride ion is surrounded by six sodium ions. Therefore, the coordination number for both Na+ and Cl- in NaCl is 6.
- Additionally, it is determined by the relative sizes and charges of the ions and is a critical factor in the overall structure of the crystal lattice in ionic compounds.
Ionic Radius:
- It is the size of an ion, which can be either a cation (positively charged) or an anion (negatively charged). It is influenced by the number of electrons, the nuclear charge, and the overall electron configuration of the ion.
- In general, cations are smaller than their parent atoms because they have lost one or more electrons, leading to a stronger effective nuclear charge that attracts the remaining electrons more closely. Anions are usually larger than their parent atoms because they have gained electrons, resulting in increased electron-electron repulsion and a larger electron cloud.
- The size of it is an essential factor in determining the coordination number in ionic compounds. A smaller ion can fit more surrounding ions, leading to a higher coordination number.
Relationship between Coordination Number and Ionic Radius:
- In ionic compounds, there is often a relationship between the coordination number and the ionic radius of the ions involved. Larger ions (with a higher ionic radius) typically have a higher coordination number because they can accommodate more surrounding ions.
- Smaller (with a smaller ionic radius) tend to have lower coordination numbers because they cannot accommodate as many surrounding ions due to their smaller size.
- This relationship between coordination number and ionic radius is crucial in understanding the crystal structures and properties of various compounds.
High Bond Energy
Definition:
Bond energy, also known as bond dissociation energy, is the energy required to break a chemical bond in a molecule, leading to the formation of separate atoms or ions. It is typically measured in units of energy per mole, such as kilojoules per mole (kJ/mol).
Factors Affecting Bond Energy:
- The bond energy of a particular bond depends on several factors, including:
- The types of atoms involved: Different elements form bonds with varying strengths. For example, a covalent bond between two oxygen atoms (O=O) is stronger than a covalent bond between two hydrogen atoms (H-H).
- The number of bonds between atoms: Double and triple bonds (e.g., C=C or N≡N) are generally stronger and have higher bond energies than single bonds.
- The bond length: The distance between the bonded atoms can influence bond strength. Shorter bonds are typically stronger.
High Bond Energy and Stability:
- Chemical compounds with high bond energies are generally more stable because they require a significant amount of energy to break their bonds. Stable compounds tend to be less reactive.
- Importance in Chemical Reactions:
- Bond energy is a critical concept in understanding chemical reactions. Reactions involve the breaking of existing bonds and the formation of new bonds. The difference in bond energies between reactants and products determines whether a reaction is exothermic (releases energy) or endothermic (absorbs energy).
- Examples:
- In the case of the diatomic molecule oxygen (O2), breaking the O=O bond requires a relatively large amount of energy, which is why oxygen is stable and doesn’t readily react with other substances.
- In hydrocarbon fuels like methane (CH4), the carbon-hydrogen (C-H) bonds have high bond energies, contributing to the energy content of these fuels.
- Applications:
- Understanding bond energy is essential in fields such as thermodynamics, combustion, and chemical engineering. It helps in the design of chemical processes, the calculation of reaction enthalpy, and the development of new materials.
- Measurement:
- Bond energies are experimentally determined using various techniques, including spectroscopy and calorimetry. These measurements provide valuable data for understanding the properties of chemical compounds.
The Role of Heat Energy
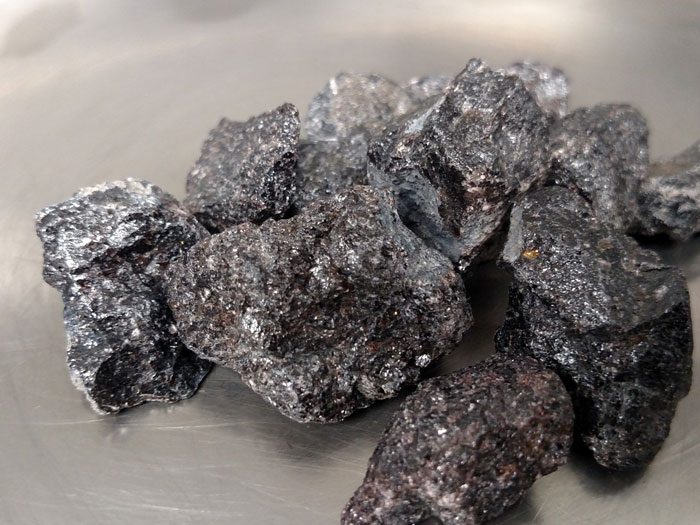
Heat energy known as heat is essential in understanding many everyday processes. It’s linked to how hot or cold something is. When we add heat to a system, it makes the tiny particles inside it move faster, and this makes things warmer.
Furthermore, it is crucial when things change from solids to liquids (like ice melting), or when they turn into gases (like water evaporating). It’s also vital in chemical reactions, where some reactions need heat, and others give off heat.
Then, We use tools like calorimeters to measure how much it is involved. Think about it as the energy that warms things up and makes stuff happen, like cooking, keeping our homes comfortable, and even how engines work. It’s an everyday concept with a big impact, both in our lives and on the planet’s climate.
FAQs
Why is aluminum oxide’s melting point high?
Aluminum oxide has a high melting point due to strong ionic and covalent bonds between its aluminum and oxygen atoms.
Why does aluminum oxide have a high boiling point?
Aluminum oxide’s high boiling point is also attributed to its strong chemical bonds, requiring substantial energy to break.
Does aluminum oxide have a high melting point?
Yes, aluminum oxide has a high melting point, typically around 2,000 to 2,200 degrees Celsius.
Why does aluminum oxide have a higher melting point than sodium oxide?
Aluminum oxide has stronger ionic and covalent bonds than sodium oxide, leading to a higher melting point.
Why does aluminum oxide have a low melting point?
Aluminum oxide is known for its high melting point, not a low one.
Why does aluminum oxide have a low melting point?
This is not accurate; aluminum oxide has a high melting point.
Does Al2O3 have a low melting point?
No, aluminum oxide (Al2O3) has a high melting point.
Why is the melting point of aluminum oxide higher than sodium chloride?
The higher melting point of aluminum oxide is due to stronger chemical bonds compared to sodium chloride.
Final Words
To cover up, the high melting point of aluminum oxide is a result of its robust atomic structure held together by strong ionic and covalent bonds. These bonds create a sturdy, three-dimensional lattice that demands a significant amount of heat energy to break.
Moreover, this property makes aluminum oxide a valuable material for applications where exceptional heat resistance is required, such as in the production of refractories, ceramics, and materials for high-temperature environments. Its remarkable melting point is a testament to the strength of its atomic bonds and its ability to withstand extreme temperatures.